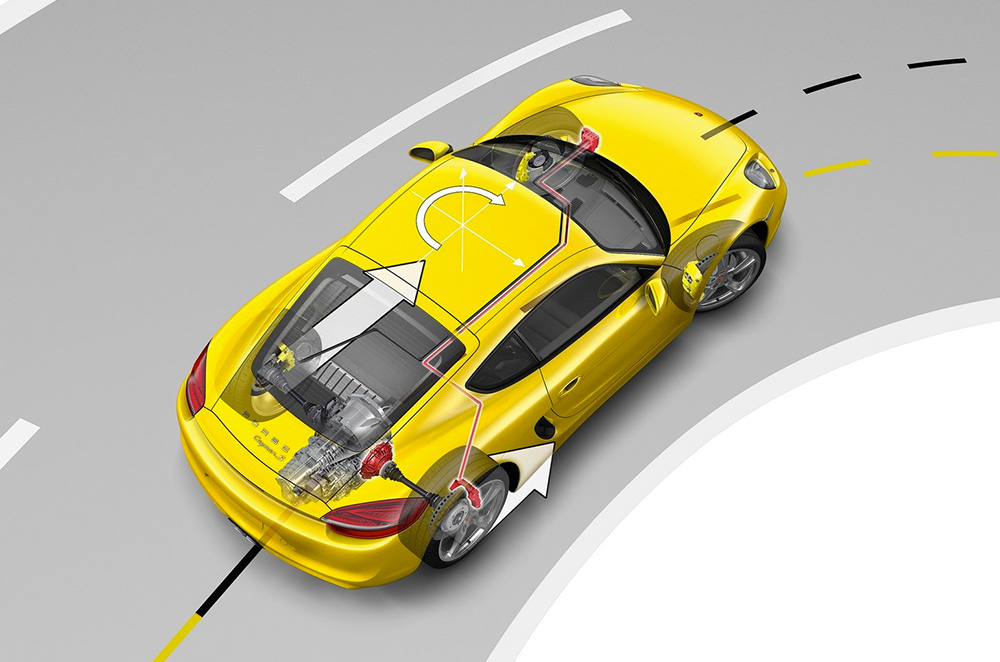
In the world of automobiles, every turn of the wheel is a dance between power and control. As technology continues to push the boundaries of what's possible on the road, a captivating choreography known as torque vectoring takes center stage. Imagine a ballet of forces, a symphony of mechanics, and a harmony of algorithms—all working together to elevate the driving experience to new heights of excitement and safety.
At this point in time, you might have read in a car brochure somewhere that torque vectoring is included in your vehicle. From the Mitsubishi Xpander Cross’ Active Yaw Control (AYC) to Mazda’s G-Vectoring Control, we are here to explain what torque vectoring really is and why it isn’t some type of marketing jargon.
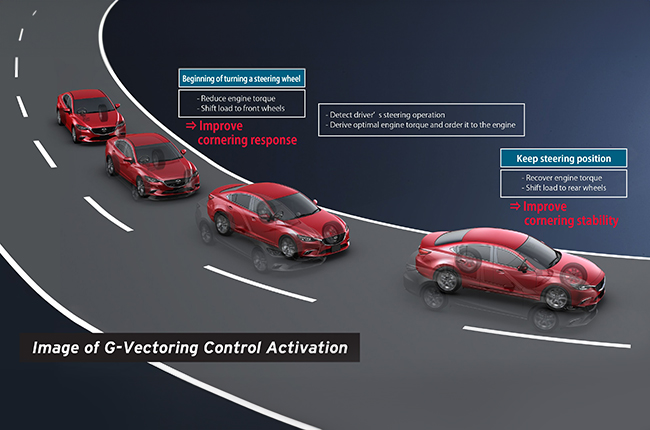
Before we can truly grasp the magic of torque vectoring, we must first understand the essence of torque itself. In simple terms, torque refers to the twisting force an engine generates, typically measured in pound-feet (lb-ft) or Newton-meters (Nm). It is the rotational force that propels the wheels forward, allowing vehicles to overcome inertia and climb slopes with ease.
Traditionally, power was distributed equally to all wheels, offering a simplistic and somewhat limited approach to traction and handling. This approach often resulted in understeer or oversteer during cornering, where the car tends to drift wide or tuck in excessively. To overcome these challenges, automotive engineers devised an ingenious solution—torque vectoring.
Although the concept of torque vectoring had been simmering in the automotive industry for decades, especially with all-wheel drive (AWD) World Rally Championship (WRC) motorsport cars and homologation vehicles, its true potential came to fruition in recent years. The dawn of electronic stability control (ESC) and advancements in computer processing opened doors to a new era of vehicle dynamics.
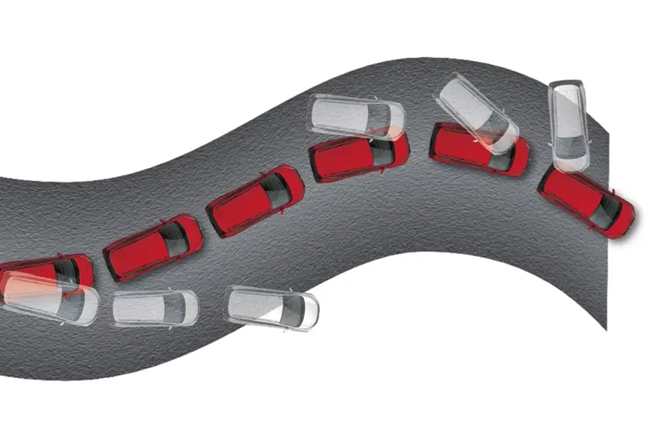
Torque vectoring represents a departure from conventional differential systems, which passively distribute power based on resistance. Instead, it involves actively managing the torque to each wheel, precisely adjusting the amount of power delivered in real-time, depending on various factors such as steering input, wheel speed, and vehicle dynamics.
Torque vectoring employs various technologies and methodologies to manipulate the vehicle's movement masterfully. Among these, two of the most prevalent systems are brake-based torque vectoring and torque-vectoring differentials.
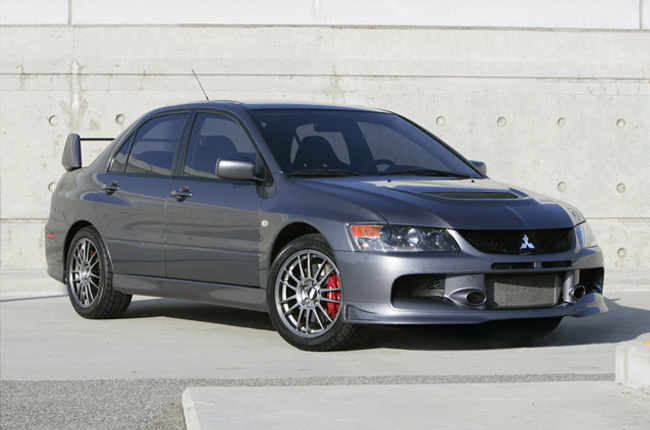
Brake-based torque vectoring involves selectively applying the brakes to individual wheels during cornering. By slowing down the inner wheel slightly, it creates a momentary imbalance, causing more torque to be transferred to the outer wheel. This process helps the vehicle turn more sharply, reducing understeer and enhancing overall stability. The electronic brain orchestrating this dance continuously monitors sensor inputs and adjusts the brake pressure seamlessly, ensuring a graceful performance on any road.
Taking a more mechanical route, torque-vectoring differentials use a set of gears or clutches to manage the distribution of torque between the left and right wheels. These differentials can be active or passive, with the active variants employing sophisticated electronic controls to optimize torque distribution actively.
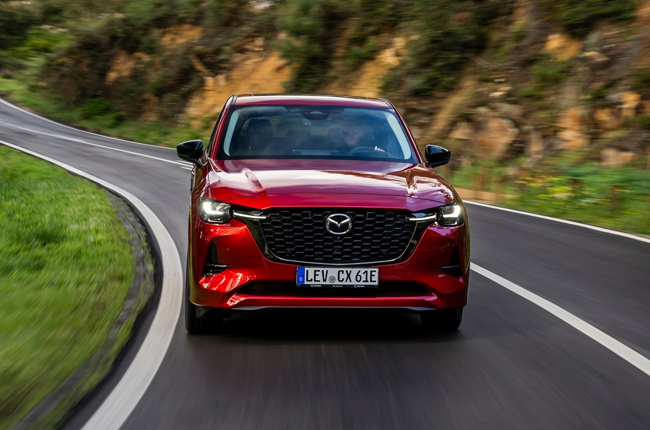
At the heart of torque vectoring lies a symphony of algorithms and sensors that interpret the driver's intentions and the vehicle's dynamic state. From steering angle and wheel speed to yaw rate and lateral acceleration, an array of sensors feed data to the central electronic control unit (ECU). The ECU, armed with proprietary algorithms and machine learning capabilities, processes this data and orchestrates the optimal torque distribution strategy.
This intelligent manipulation of torque enhances not only the car's agility and cornering abilities but also its safety. By intelligently redistributing power, torque vectoring can mitigate the risk of oversteer or understeer, preventing potential loss of control in challenging driving conditions.
To explain this in a more laid-back manner, when taking a corner hard, the vehicle wants to drag you inside the corner more, hence reducing understeer, especially for front-wheel-drive (FWD) and all-wheel-drive cars (AWD).
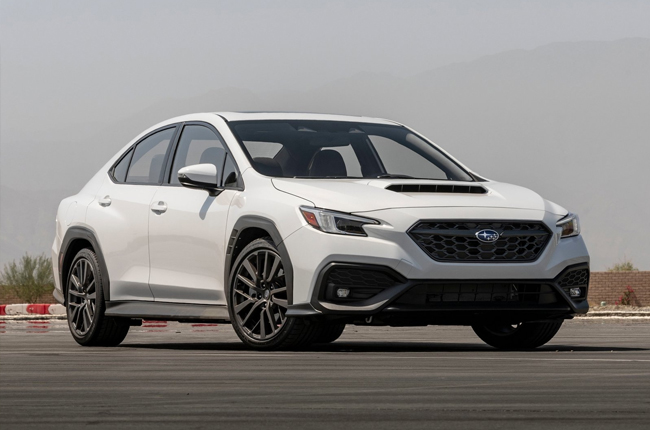
The rise of torque vectoring has left an indelible mark on the driving experience, redefining the limits of performance and safety. In performance-oriented vehicles, torque vectoring allows drivers to carve corners with surgical precision, inducing a sense of connection and excitement previously reserved for racing circuits.
Beyond the thrill of spirited driving, torque vectoring has also had a profound impact on safety. By fine-tuning the power delivery to each wheel, the system can enhance stability and grip, especially in adverse weather conditions or emergency maneuvers. This technology has revolutionized the world of driving making them more than just a tool for high-speed rallying but also a means to elevate on-road confidence and control on the tarmac.
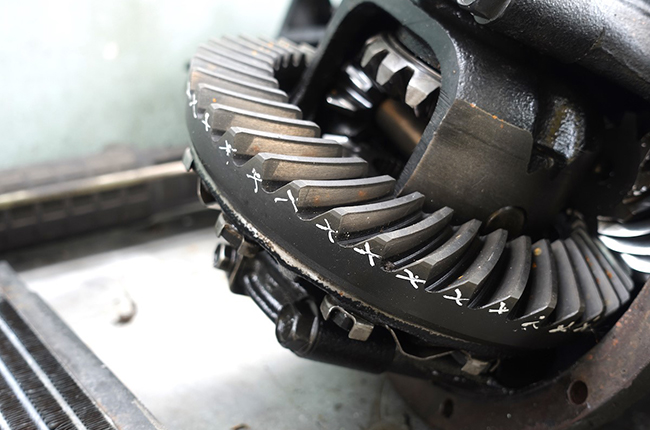
All in all, it’s great to see this technology trickle down to regular road cars, if it means added safety, and more importantly, added fun in the corners.
Latest Features
-
Should the 2024 Nissan Livina VE be your next family car? / Featured Article
The Nissan Livina VE is here to turn your family trips into good memories with its features and design.
-
Which Ford should I buy? / Tips & Advice
What Ford will be yours?
-
Top 8 overland-capable vehicles available in the Philippines / Featured Article
These overland-capable vehicles sold in the Philippines are our pick to jump-start your adventure.
Popular Articles
-
Cheapest cars under P700,000 in the Philippines
Aug 25, 2023
-
First car or next car, the Ford EcoSport is a tough package to beat
Jun 18, 2021
-
Car Maintenance checklist and guide – here’s everything you need to know
Earl Lee · Jan 12, 2021
-
Most fuel efficient family cars in the Philippines
Bryan Aaron Rivera · Nov 27, 2020
-
2021 Geely Okavango — Everything you need to know
Joey Deriquito · Nov 19, 2020
-
Family cars in the Philippines with the biggest trunks
Sep 20, 2023
-
Head to head: Toyota Rush vs. Suzuki XL7
Joey Deriquito · Oct 28, 2020
-
Why oil changes are important for your car
Earl Lee · Nov 10, 2020
-
2021 Kia Stonic — What you need to know about it
Joey Deriquito · Oct 16, 2020
-
Top 7 tips for buying a used car in the Philippines
Joey Deriquito · Nov 26, 2020